All about the sun?
The Sun, like all stars, is an enormous ball of extremely hot, largely ionized gas, shining under its own power. And we do mean enormous. The Sun could fit 109 Earths side-by-side across its diameter, and it has enough volume (takes up enough space) to hold about 1.3 million Earths.
The Sun does not have a solid surface or continents like Earth, nor does it have a solid core (Figure 1). However, it does have a lot of structure and can be discussed as a series of layers, not unlike an onion. In this section, we describe the huge changes that occur in the Sun’s extensive interior and atmosphere, and the dynamic and violent eruptions that occur daily in its outer layers.
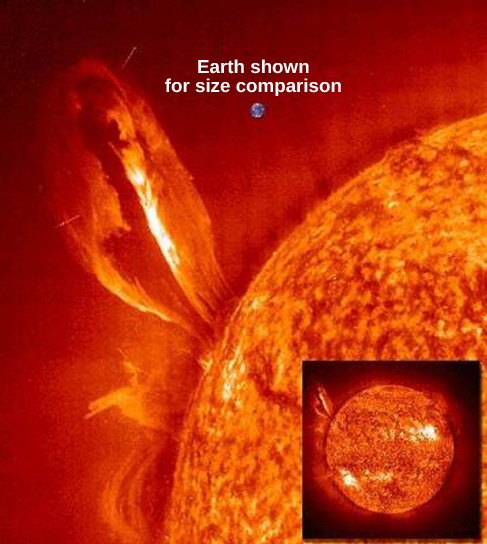
Figure 1. Earth and the Sun: Here, Earth is shown to scale with part of the Sun and a giant loop of hot gas erupting from its surface. The inset shows the entire Sun, smaller. (credit: modification of work by SOHO/EIT/ESA)
Some of the basic characteristics of the Sun are listed in Table 1. Although some of the terms in that table may be unfamiliar to you right now, you will get to know them as you read further.
Table 1. Characteristics of the Sun | ||
---|---|---|
Characteristic | How Found | Value |
Mean distance | Radar reflection from planets | 1 AU (149,597,892 km) |
Maximum distance from Earth | 1.521 × 108 km | |
Minimum distance from Earth | 1.471 × 108 km | |
Mass | Orbit of Earth | 333,400 Earth masses (1.99 × 1030 kg) |
Mean angular diameter | Direct measure | 31´59´´.3 |
Diameter of photosphere | Angular size and distance | 109.3 × Earth diameter (1.39 × 106 km) |
Mean density | Mass/volume | 1.41 g/cm3
(1400 kg/m3)
|
Gravitational acceleration at photosphere (surface gravity) | GM/R2 | 27.9 × Earth surface gravity = 273 m/s2 |
Solar constant | Instrument sensitive to radiation at all wavelengths | 1370 W/m2 |
Luminosity | Solar constant × area of spherical surface 1 AU in radius | 3.8 × 1026 W |
Spectral class | Spectrum | G2V |
Effective temperature | Derived from luminosity and radius of the Sun | 5800 K |
Rotation period at equator | Sunspots and Doppler shift in spectra taken at the edge of the Sun | 24 days 16 hours |
Inclination of equator to ecliptic | Motions of sunspots | 7°10´.5 |
Composition of the Sun’s Atmosphere
Let’s begin by asking what the solar atmosphere is made of. As explained in Radiation and Spectra, we can use a star’s absorption line spectrum to determine what elements are present. It turns out that the Sun contains the same elements as Earth but not in the same proportions. About 73% of the Sun’s mass is hydrogen, and another 25% is helium. All the other chemical elements (including those we know and love in our own bodies, such as carbon, oxygen, and nitrogen) make up only 2% of our star. The 10 most abundant gases in the Sun’s visible surface layer are listed in Table 1. Examine that table and notice that the composition of the Sun’s outer layer is very different from Earth’s crust, where we live. (In our planet’s crust, the three most abundant elements are oxygen, silicon, and aluminum.) Although not like our planet’s, the makeup of the Sun is quite typical of stars in general.
Table 1. The Abundance of Elements in the Sun | ||
---|---|---|
Element | Percentage by Number of Atoms | Percentage By Mass |
Hydrogen | 92.0 | 73.4 |
Helium | 7.8 | 25.0 |
Carbon | 0.02 | 0.20 |
Nitrogen | 0.008 | 0.09 |
Oxygen | 0.06 | 0.80 |
Neon | 0.01 | 0.16 |
Magnesium | 0.003 | 0.06 |
Silicon | 0.004 | 0.09 |
Sulfur | 0.002 | 0.05 |
Iron | 0.003 | 0.14 |
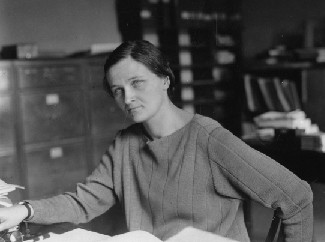
Figure 2. Cecilia Payne-Gaposchkin (1900–1979): Her 1925 doctoral thesis laid the foundations for understanding the composition of the Sun and the stars. Yet, being a woman, she was not given a formal appointment at Harvard, where she worked, until 1938 and was not appointed a professor until 1956. (credit: Smithsonian Institution)
The fact that our Sun and the stars all have similar compositions and are made up of mostly hydrogen and helium was first shown in a brilliant thesis in 1925 by Cecilia Payne-Gaposchkin, the first woman to get a PhD in astronomy in the United States (Figure 2). However, the idea that the simplest light gases—hydrogen and helium—were the most abundant elements in stars was so unexpected and so shocking that she assumed her analysis of the data must be wrong. At the time, she wrote, “The enormous abundance derived for these elements in the stellar atmosphere is almost certainly not real.” Even scientists sometimes find it hard to accept new ideas that do not agree with what everyone “knows” to be right.
Before Payne-Gaposchkin’s work, everyone assumed that the composition of the Sun and stars would be much like that of Earth. It was 3 years after her thesis that other studies proved beyond a doubt that the enormous abundance of hydrogen and helium in the Sun is indeed real. (And, as we will see, the composition of the Sun and the stars is much more typical of the makeup of the universe than the odd concentration of heavier elements that characterizes our planet.)
Most of the elements found in the Sun are in the form of atoms, with a small number of molecules, all in the form of gases: the Sun is so hot that no matter can survive as a liquid or a solid. In fact, the Sun is so hot that many of the atoms in it are ionized, that is, stripped of one or more of their electrons. This removal of electrons from their atoms means that there is a large quantity of free electrons and positively charged ions in the Sun, making it an electrically charged environment—quite different from the neutral one in which you are reading this text. (Scientists call such a hot ionized gas a plasma.)
In the nineteenth century, scientists observed a spectral line at 530.3 nanometers in the Sun’s outer atmosphere, called the corona (a layer we will discuss in a minute.) This line had never been seen before, and so it was assumed that this line was the result of a new element found in the corona, quickly named coronium. It was not until 60 years later that astronomers discovered that this emission was in fact due to highly ionized iron—iron with 13 of its electrons stripped off. This is how we first discovered that the Sun’s atmosphere had a temperature of more than a million degrees.
The layer of the sun beneath the surface
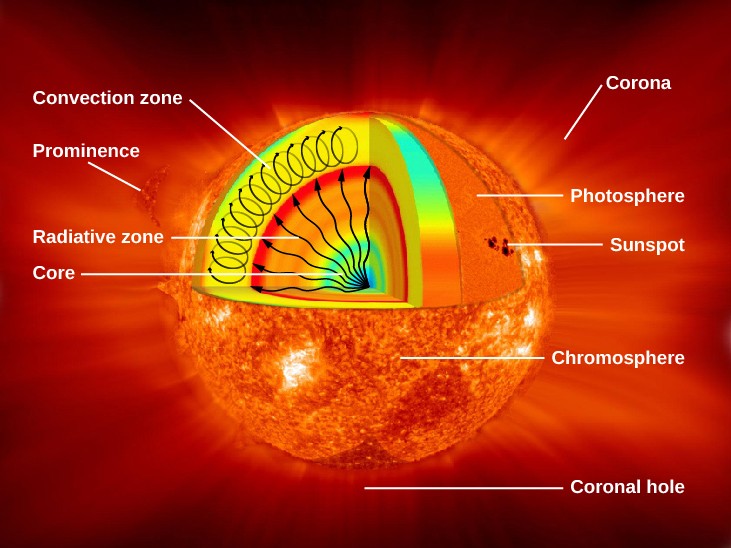
Figure 3. Parts of the Sun: This illustration shows the different parts of the Sun, from the hot core where the energy is generated through regions where energy is transported outward, first by radiation, then by convection, and then out through the solar atmosphere. The parts of the atmosphere are also labeled the photosphere, chromosphere, and corona. Some typical features in the atmosphere are shown, such as coronal holes and prominences. (credit: modification of work by NASA/Goddard)
Figure 3 shows what the Sun would look like if we could see all parts of it from the center to its outer atmosphere; the terms in the figure will become familiar to you as you read on.
The Sun’s layers are different from each other, and each plays a part in producing the energy that the Sun ultimately emits. We will begin with the core and work our way out through the layers. The Sun’s core is extremely dense and is the source of all of its energy. Inside the core, nuclear energy . The core is approximately 20% of the size of the solar interior and is thought to have a temperature of approximately 15 million K, making it the hottest part of the Sun.
Above the core is a region known as the radiative zone—named for the primary mode of transporting energy across it. This region starts at about 25% of the distance to the solar surface and extends up to about 70% of the way to the surface. The light generated in the core is transported through the radiative zone very slowly, since the high density of matter in this region means a photon cannot travel too far without encountering a particle, causing it to change direction and lose some energy.
The convective zone is the outermost layer of the solar interior. It is a thick layer approximately 200,000 kilometers deep that transports energy from the edge of the radiative zone to the surface through giant convection cells, similar to a pot of boiling oatmeal. The plasma at the bottom of the convective zone is extremely hot, and it bubbles to the surface where it loses its heat to space. Once the plasma cools, it sinks back to the bottom of the convective zone.
Now that we have given a quick overview of the structure of the whole Sun, in this section, we will embark on a journey through the visible layers of the Sun, beginning with the photosphere—the visible surface
0 Comments